Inicio » Medicine preparation (Página 2)
Archivo de la categoría: Medicine preparation
Atoms and Bonding
Living things are unique among all forms of matter.
Unlike non-living things, all living things — from single-celled organisms such as the Euglena in Figure 1.1 to multicelled organisms such as whales and redwood trees — interact with and manipulate matter and energy. For example, all cells take in essential substances such as oxygen, water, and nutrients from their external environment. Inside cells, these substances undergo chemical reactions of several types. These reactions may be used to
break down substances, synthesize others, and repair defective structures. Chemical reactions also provide energy for these life-sustaining activities, as well as others such as reproduction. Unneeded (or harmful) products of the reactions are eliminated as wastes.
Collectively, these processes — intake of substances, processing of substances, and elimination of wastes — are called metabolic processes, or metabolism. The substances involved in metabolism are molecules. The bonds that form
between atoms define the structure and properties of these molecules. In this section, you will review several key ideas about atoms and bonding.

Figure 1.1 Euglena, a unicellular freshwater organism, carries out the same metabolic processes that your cells do.
Atoms and Elements
As you have learned in previous studies, all matter is formed of atoms. The atom is the smallest unit of matter involved in chemical reactions. Although tiny, atoms are complex structures, composed of even smaller subatomic particles. Most students of chemistry still study the model of the atom that Danish physicist Niels Bohr presented in the early twentieth century (see Figure 1.2). In this model, an atom consists of a small, dense core called a nucleus. It is composed of two kinds of subatomic particles — the positively (+) charged protons and the uncharged, or neutral, neutrons. Also in the Bohr model, negatively (−) charged electrons orbit the nucleus in one or more energy levels, or shells.

Figure 1.2 Niels Bohr’s model of the neon atom
An element is a substance that cannot be broken down into simpler substances by chemical means. Substances such as calcium, oxygen, potassium, iron, and carbon are all elements. A few elements, such as helium, occur as single atoms. Several elements, such as hydrogen, nitrogen, and oxygen, occur as molecules made up of two atoms. Such molecules are called diatomic. Other elements such as phosphorus and sulfur occur as molecules made up of more than two atoms.
All atoms of an element have the same number of protons in their nuclei. This number, called the atomic number, is different for every element. The nuclei of carbon atoms, for example, each contain six protons. Because the nuclei of most atoms also contain neutrons, another important characteristic of an atom is its mass number. The mass number of an atom is the total number of protons and neutrons in its nucleus. Atoms of the same element that contain different numbers of neutrons are called isotopes of that element. Refer to Figure 1.3 to see the numbers of protons, neutrons, and electrons in three isotopes of carbon. Their names include the mass number of each isotope: carbon-12, carbon-13, and carbon-14.

Figure 1.3 Carbon, one of the most important elements in living matter, has three naturally occurring isotopes. The
nucleus of each isotope contains 6 protons, but the number of neutrons in the nucleus is 6, 7, or 8. In each isotope,
6 electrons exist outside the nucleus.
Some isotopes are stable, whereas others are unstable and break down (decay). The unstable isotopes are known as radioactive isotopes.
Carbon-12 and carbon-13 are both stable isotopes, whereas carbon-14 is unstable and decays. Many radioactive isotopes decay at known rates. The rate at which a radioactive isotope decays may be used scientifically. The decay of carbon-14 can be used by archeologists, in a process called radiocarbon dating, to find the ages of some objects up to about 50 000 years old.

Table 1.1 shows the atomic masses of the elements that are most abundant in living organisms. Notice that, unlike atomic numbers and mass numbers, some atomic masses are not whole numbers. This is the case because the atomic mass of an element is the average mass of all the naturally occurring isotopes of that element.
Chlorine, for example, naturally occurs as a mixture of two isotopes: chlorine-35 and chlorine- 37. There are three chlorine-35 atoms for every chlorine-37 atom. Therefore, the average mass of chlorine atoms is closer to 35 than to 37. The atomic mass of chlorine is, in fact, 35.5 u (atomic mass units). Appendix 7 provides atomic masses for all the known elements.
Electron Energy
Biologists usually study the groups of atoms that make up molecules rather than atoms and subatomic particles themselves. All cells obtain the energy to function from chemical reactions that involve molecules. The actions of electrons are key to this process.
According to the Bohr model, electrons orbit the nucleus of an atom within energy levels, or shells. An electron in the first shell (nearest the nucleus) has the lowest amount of potential energy. Any electrons in the remaining shells have more potential energy. Each shell can hold a maximum number of electrons. The first shell, for example, can hold a maximum of two electrons, while the second shell can hold a maximum of eight. Refer to Figure 1.2, which shows that in a neon atom the first two shells are filled. In general, the maximum number of electrons that a shell can hold is given
by the formula 2n2, where n is the number of the shell. For example, the third shell can hold a total of 2(3)2 = 18 electrons.
The chemical properties of atoms rely mostly on the number of electrons in the outermost, occupied shell of an atom in its lowest energy state. This shell is known as the valence shell. The electrons that occupy the valence shell of any atom are called valence electrons. The elements in the periodic table that are least reactive are the noble gases, such as neon, found in group 18(8A) (see Appendix 7). Atoms of the other elements in the periodic table are more reactive than the noble gases. These elements can form chemical bonds with each other. The MiniLab examines safety issues involving the use of chemicals and how they react with each other during chemical bonding.
Ionic and Covalent Bonds
Most atoms can form chemical bonds with other atoms. These bonds are the forces that hold the atoms together in the form of compounds. For example, two chlorine atoms can combine (chemically react) to form a diatomic molecule of the element chlorine (Cl2). Atoms of sodium and chlorine can combine to form the ionic compound sodium chloride (NaCl).
There are two general types of chemical bonds. One type involves the sharing of electrons between atoms, and is known as a covalent bond. The other type involves the transferring of one or more electrons from one atom to another, and is called an ionic bond. How are these bonds formed?

Any atom has the same number of electrons and protons. Therefore, the atom has no charge and is said to be neutral. However, if an atom loses or gains electrons, that atom becomes an ion. If an atom loses electrons, the ion formed has more protons than electrons and therefore has a positive charge. A positively charged ion is called a cation.
In contrast, if an atom gains one or more electrons the ion formed has a negative charge. A negatively charged ion is called an anion. When sodium (Na) and chlorine (Cl) atoms react, they form an ionic bond, as shown in Figure 1.4. The sodium atom gives up its only valence electron and becomes a sodium ion, with 11 protons and 10 electrons. This
number of electrons is arranged in the same way that the 10 electrons are arranged in the neon atom.
The chlorine atom gains an electron and becomes a chloride ion, with 17 protons and 18 electrons. This number of electrons is arranged in the same way as the 18 electrons in an argon (Ar) atom. Because the sodium ion is positively charged and the chloride ion is negatively charged, they attract each other to form an ionic bond. The tendency of chlorine to gain electrons is characteristic of atoms with a few electrons less than a noble gas atom. For example, atoms of fluorine and oxygen also tend to gain electrons when they form ionic bonds. One way to understand which elements form ionic bonds when they react is to use the principle of electronegativity.
Electronegativity is a measure of the relative abilities of bonding atoms to attract electrons. The Pauling scale is the most commonly used measure of electronegativities of atoms. Fluorine, the most electronegative element, is found near the top right corner of the periodic table and has an electronegativity value of 4.0. Both cesium and francium, the least electronegative elements, are found near the bottom left corner of the periodic table and each has an electronegativity value of 0.7. Elements that are most likely to form ionic bonds, such as sodium and chlorine, are far apart in the periodic table and have a large difference in their electronegativity. Elements that are close together in the
periodic table have a small difference in their electronegativity. If two of these elements react to form a compound, their similar abilities to attract electrons results in the formation of a covalent bond, in which electrons are shared. In a covalent bond, atoms share two valence electrons. An example of this is the covalent bonding of two chlorine atoms, as shown in Figure 1.5, top. Double covalent bonds involve the sharing of two pairs of shared valence electrons. The two oxygen atoms in an oxygen molecule are joined by a double covalent bond, as shown in Figure 1.5, middle.

The shared electrons in covalent bonds belong exclusively to neither one nor the other atom. However, by sharing these valence electrons, both atoms appear to have the same number of valence electrons as a noble gas atom. In a covalent bond formed by two atoms of the same element, the electronegativity difference is zero. Therefore, the
electrons in the bond are shared equally between the two atoms. This type of bond is described as non-polar covalent. Examples of non-polar covalent bonds are found in chlorine and carbon dioxide molecules. A covalent bond is said to be polar covalent when the electronegativity difference between the atoms is not zero and the electrons are therefore shared unequally. In a water molecule (see Figure 1.5, bottom), oxygen is more electronegative than is hydrogen. The shared electrons spend more of their time near the oxygen nucleus than near the hydrogen nucleus. As a result, the oxygen atom gains a slight negative charge and the hydrogen atoms become slightly positively charged.
Chemists represent molecules formed through covalent bonds with various formulas, such as those in Figure 1.6. Electron-dot and structural formulas are simplified ways of showing what electrons are being shared.

Hydrogen bonds and the properties of water
Some molecules with polar covalent bonds are known as polar molecules. A polar molecule has an unequal distribution of charge as a result of its polar bonds and its shape. More information about polar molecules is provided in Appendix 6. Water is a common example of a polar molecule. In a water molecule, as shown in Figure 1.7, the slightly negative end of each bond can be labelled δ− and the slightly positive end can be labelled δ+. These two ends, with slightly different charges, are sometimes referred to as “poles.” Because a water molecule is polar, it can attract other water molecules, due to the attraction between negative poles and positive poles (see Figure 1.7). The attractions between water molecules are called hydrogen bonds. Hydrogen bonds can also be found between other molecules that contain hydrogen atoms bonded covalently to atoms of a much more electronegative element. Examples include ammonia (NH3) and hydrogen fluoride (HF) in their liquid states.

A hydrogen bond is a force between molecules, not a chemical bond within a molecule. Hydrogen bonds are usually weaker than chemical bonds. For instance, a hydrogen bond may be only five percent the strength of a covalent bond, but it is sufficient to hold one water molecule to another in liquid water or ice. Under normal conditions, water molecules are attracted to each other in such a way that they are neither attracted too strongly (to form a solid) nor too weakly (allowing water to become a vapour). For this reason, under normal conditions on Earth, water exists as a liquid.
Solubility of Substances in Water
All cells depend on liquid water. In fact, living organisms contain more molecules of water than any other substance; water comprises as much as 90 percent of a typical cell. Water is a perfect fluid environment through which other molecules can move and interact. Sodium chloride (table salt), and many other ionic compounds or salts, dissolve readily in water. This occurs because the positively charged poles of the water molecule are attracted to the anions
(chloride ions) in the salt. The negatively charged pole of the water molecule is similarly attracted to the cations (sodium ions) in the salt as shown in Figure 1.8. These two attractions pull the sodium ions and chloride ions away from each other. The salt is now dissociated, which means that the sodium ions and chloride ions have separated and have dissolved in the water.

Compounds that interact with water — for example, by dissolving in it — are called hydrophilic. In contrast, compounds that do not interact with water are called hydrophobic. Nonpolar compounds are hydrophobic. They cannot form hydrogen bonds with water in the same way that ionic or polar compounds can. Therefore, hydrophobic molecules are insoluble in water. For example, when you place a drop of oil (a non-polar compound of carbon and hydrogen) into water, the oil does not mix with the water — they remain separate.
In this section, you have learned that the type of chemical bond that joins individual atoms together determines whether the resulting compound is ionic or covalent. Covalent molecules may be polar or non-polar, depending on the electronegativities of the bonded atoms and shape of the molecule.
You have learned that hydrogen bonds form between molecules in water, which interacts very differently with hydrophobic and hydrophilic compounds. Hydrophobic interactions especially have a great effect on many biological molecules. For instance, many protein molecules have hydrophobic regions in portions of their structure.
Interactions of these regions with water cause the molecules to adopt specific shapes. You will see examples of this in the next section, which reviews the four main kinds of molecules that make up all cells.
TRANSPORT IN PLANTS AND ANIMALS
Introduction
The movement of materials within the body tissues or between tissues in multicellular organisms like high plants and animals occurs in transport systems. In unicellular or low plants and animals, such movement is by the transport mechanisms of molecules within a transport medium by diffusion, osmosis, active transport and mass flow, among others.
In multicellular animals, the medium of transport is blood, while in plants, it is water in which the materials are dissolved and transported. In case of diseases such as diarrhoea, dysentery, malaria, etc, the animal body may
undergo dehydration. This causes serious effects on the body’s physiological process, which can lead to death. Just like dehydration in animals, in plants wilting or excess water loss results, especially during dry seasons or inadequate water supply or absorption by conducting tissues.
Animals and plants have specialised cells or tissues performing special functions of the transport systems apart from transporting materials.
Discuss the role of protection and support of the body structure, by the transport systems in animals and plants.
The major significance of these systems to the body has been greatly applied to economic development, for example by animal health service providers, in the construction industry using plant materials and in environmental
protection.
Background information
Transport involves the movement of materials from one part of the organism to another. In animals, it is basically the circulatory system. The system consists of the heart and blood vessels. Blood flow through the system is maintained by the heart, skeletal muscles, valves, and inspiratory movements. The heart is myogenic. Blood transports dissolved substances such as respiratory gases, water, food materials, etc.
In case of diseases such as malaria, diarrhoea and others, the body may lose blood together with water, leading to dehydration. This causes serious effects including dizziness and even death. This condition can be corrected
by administering dalose.
Explanatory note
Oral rehydration mixtures are used to boost the amount of water lost from the
body due to dehydration. Dehydration is a condition developed in patients
that have experienced illness, leading to low water amounts in blood fluid.
Rehydration helps to add water to the body so that transport of materials in
the body is efficient.
VITAL FUNCTIONS IN ANIMALS
ANIMAL NUTRITION
Nutrition in animals is heterotrophic. This means that animals feed on other living things.
Animals can be classified according to their nutrition function: herbivores (plant eaters), carnivores (eat other animals), omnivores (eat animals and plants) and saprophytic (feed on decaying organic matter).
In order to perform the vital function of nutrition, animals have different systems: digestive, respiratory, circulatory and excretory.
Digestive system
The digestive system obtains nutrients from food though the process of digestion.
In most animals, digestion takes place in the digestive tract. The digestive tract begins in the mouth, where food is torn and crushed before it is swallowed.
Next, the resulting paste goes through the oesophagus, stomach and intestines. This is where digestion is completed, and nutrients pass into the blood.
The digestive tract ends in the anus. It is through this organ that unabsorbed food is ejected though the process of defecation.
Throughout the digestive tract, there are organs called glands. They produce substances that help break down food into simpler molecules.
Respiratory system
The respiratory system obtains oxygen (O2) from the environment and releases carbon dioxide (CO2) that has accumulated in the cells.
Almost all animals have specialised organs dedicated to breathing, except for a few very simple animals.
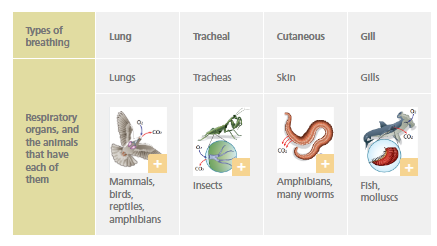
Circulatory system
The circulatory system takes nutrients absorbed during digestion to the cells, as well as the oxygen absorbed during respiration. In addition, it takes and transports waste products.
This system is composed of a liquid that travels around the organism transporting substances, vessels in which the liquid moves, and one or more hearts that pump the liquid.
There are two types of circulatory system:
- Open circulatory system: in some areas, the liquid leaves the vessels and bathes the cells directly. This type of system can be found in some invertebrates.
- Closed circulatory system: the liquid always travels inside the vessels. This type of system can be found in all vertebrate animals and in some invertebrate animals.
Excretory system
Chemical reactions in organisms produce metabolic waste substances. CO2 is expelled through the respiratory system. Nitrogen compounds that could be harmful to the organism are also released. The circulatory system collects these substances and transports them to the excretory system, which eliminates them.
Nitrogen compounds are released by specialised excretory organs: nephridiums in annelids and molluscs, Malpighian tubes in insects, and kidneys in vertebrates.
ANIMAL INTERACTION
The nervous and endocrine systems perform the interaction function. The locomotive system is also involved if the response involves movement.
The nervous and endocrine systems work together to coordinate responses to stimuli.
NERVOUS SYSTEM
Animals detect changes in the external and internal environment. Most animals have sense organs to perceive external stimuli.
The information which is perceived is converted into nerve impulses. These are transmitted by the sensory nerves to the nerve centres.
Invertebrates have nodes that function as nerve centres. In vertebrates, the nerve centres are the brain and the spinal cord.
- The brain processes the information received and prepares complex responses.
- The spinal cord produces simple responses. These are called reflex actions. It also takes the information from the sensory nerves to the brain and then, from the brain to the motor nerves.
Motor nerves take the response to the effector organs that act on it. The nervous and endocrine systems are in charge of performing the interaction function. If the response involves movement, these could be muscles, or if a secretion is involved, these could be glands.
Endocrine system
The endocrine system is made up of glands in the body which release hormones into the blood in response to certain stimuli.
Hormones act specifically on certain cells, causing them to respond.
The endocrine system is involved in processes such as metamorphosis, growth and reproductive cycles. Endocrine responses are slower than nervous responses, but they last longer.
ANIMAL REPRODUCTION
Like other living things, animals carry out different types of reproduction.
- Asexual reproduction is found in some invertebrate species in two different ways:
• Budding: a new individual will develop from a bud or bulge in the body.
• Excision and fragmentation: the animal’s body divides into two parts (excision) or into several parts (fragmentation) to create a new individual.
- Sexual reproduction is the most common type of reproduction. It takes place in the reproductive system where the sexual cells or gametes can be found:
• The testicles are the male sex organs and produce sperm.
• The ovaries are the female sex organs and form the eggs.
Some species, such as snails, are hermaphrodites. Hermaphrodite organisms have both male and female sex organs, although they usually mate with another individual to reproduce.
Gametes are joined in the process of fertilisation. This process happens either inside the female’s body (internal fertilization) or outside it (external fertilization).
After fertilisation is complete, a zygote is created which divides itself to form an embryo.
Embryonic development in animals can be: oviparous, when the embryo develops inside an egg, viviparous, if it develops inside the mother, or ovoviviparous, when it develops inside an egg that remains inside the mother’s body.
- Some invertebrates, such as certain jellyfish, display alternating reproduction.
VITAL FUNCTIONS IN PLANTS
Like all living things, plants can perform the three vital functions of nutrition, interaction and reproduction.
PLANT NUTRITION
Plants use autotrophic nutrition. In order to carry out this process, they start by taking in inorganic matter through their roots and leaves:
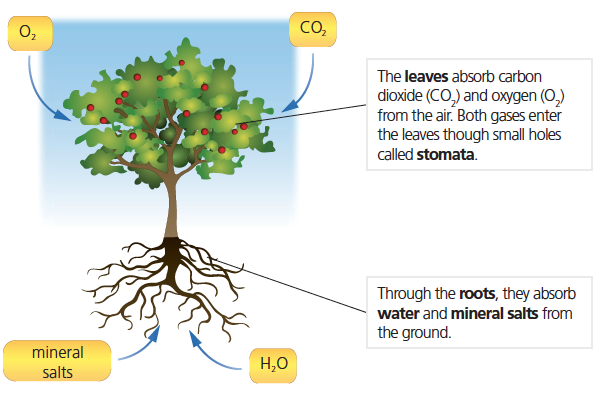
Water and the mineral salts form the raw sap which travels to the leaves through the vessels that form the xylem.
Through the process of photosynthesis, plants create organic matter from inorganic matter. This process is possible thanks to a substance called chlorophyll. It is located in the chloroplasts of plant cells, particularly in the leaves.
Chlorophyll captures energy from sunlight, and the plant uses this energy to create organic material from water, mineral salts and carbon dioxide (CO2). In this process, the plant produces oxygen (O2) and releases it into the environment.
Photosynthesis:
water + mineral salts + CO2 + sunlight g organic matter + O2
Organic matter produced during photosynthesis is shared across the whole plant as elaborated sap. This fluid travels through the vessels that make up the phloem.
The plant uses organic matter to create new structures and to perform cell respiration. This process takes place in the mitochondria. It consists of using organic matter to produce energy. During cell respiration, plants take in O2 and they release CO2.
Cell respiration:
organic matter + O2 g water + CO2 + energy
PLANT INTERACTION
Plants interact by reacting to stimuli such as light, temperature and humidity. Stimuli are also related to the growth and flowering of the plant.
Plants react to stimuli by using movement and generating hormones.
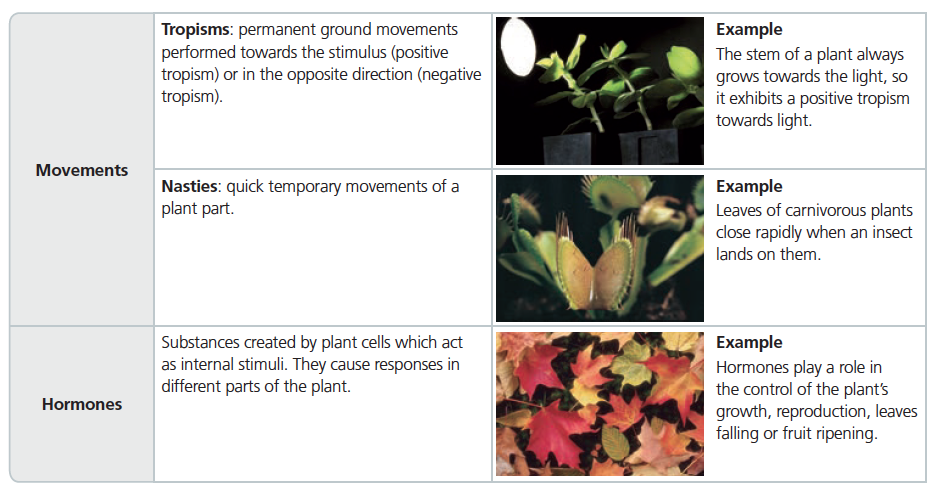
PLANT REPRODUCTION
Plants perform different types of reproduction, such as sexual, asexual or alternating.
- Asexual reproduction in plants can be exhibited in different ways:
- Sexual reproduction is characteristic of plants that produce seeds. Located in the flower are the male reproductive organs, the stamens, as well as the female reproductive organ, the carpel. The male sexual cells (pollen) are produced in the stamens, and the female ones (ovules) are produced in the carpel.
- Alternating reproduction takes place in mosses and ferns. These plants take on two different forms over the course of their life cycles: gametophyte and sporophyte. The gametophyte reproduces itself sexually by means of gametes, and the sporophyte reproduces itself asexually by means of spores.
Tasks
VITAL FUNCTIONS
In order to live, organisms use the function of nutrition to obtain the matter and energy they need.
They interact with their environment thanks to the function of interaction, and they continue to have descendants due to the function of reproduction.
NUTRITION
The purpose of nutrition is to renew and help preserve the organism’s structure as well as obtaining energy to perform the three vital functions.
Cellular metabolism is a combination of chemical reactions. This enables cells to obtain the necessary nutrients and energy to keep them alive.
In order to feed themselves, living things take substances from the environment which surrounds them, and cellular metabolism takes place in their cells.
Metabolism consists of two stages: anabolism and catabolism.
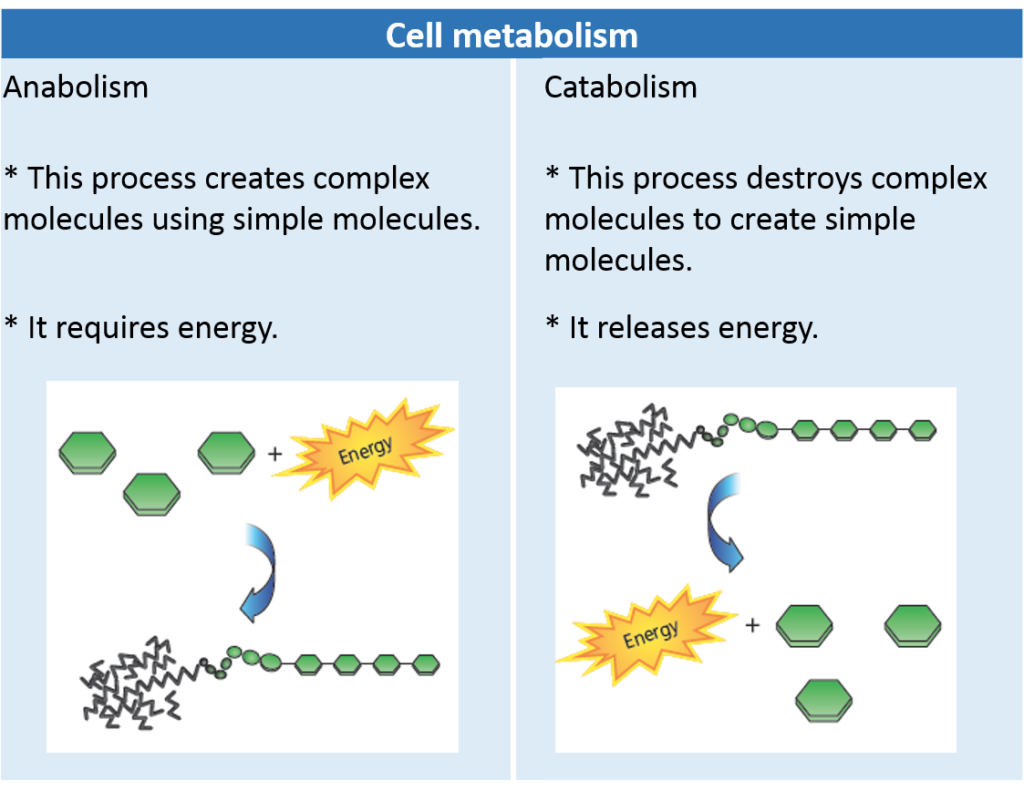
During cell metabolism, in addition to matter and energy, waste substances are released from the organism.
Nutrition types
There are two types of nutrition, depending on the type of matter obtained:
- Autotrophic nutrition: This type of nutrition is performed by organisms that obtain inorganic materials from the environment, which they transform into organic material. Plants, algae, and some bacteria perform autotrophic nutrition.
- Heterotrophic nutrition: This is performed by organisms that take organic matter from the environment. Examples of living things that use heterotrophic nutrition are: animals, fungi, protozoa and some bacteria.
INTERACTION
Interaction allows living things to interact with their environment, detect any changes in it and react to them.
Stimuli are the changes in the environment that may provoke a response in a living thing. They are perceived by the receptors, which are cells or structures specialised in perceiving stimuli.
Living things can respond to stimuli either with movements or by producing substances called hormones, which produce changes in how something functions or behaves.
REPRODUCTION
The purpose of reproduction is to create descendants and, in this way, continue the species.
There are two types of reproduction: asexual and sexual.
- Asexual reproduction: a single individual creates multiple descendants which are identical. It occurs in all unicellular organisms and in some multicellular ones. Unicellular living things only have one cell, so they can only reproduce by using the same cell.
There are three main types:
- • Bipartition: the cell divides into two cells of similar size.
- • Budding: the cell also divides into two cells, but this time they are very different in size.
- • Sporulation: one single individual divides its nucleus, creating many new individuals.
- • Sexual reproduction:it requires two individuals of opposite sex. The number of descendants is smaller than in asexual reproduction and the offspring are not identical. Multicellular organisms perform this type of reproduction.
Some species are capable of performing both types of reproduction.
ACTIVITIES
CELLS
Biomolecules group together to create cells: the smallest structures that can be considered living things.
Cells are the functional and structural units of all living things.
All living things, including human beings, are made of cells. This is why cells are structural units. At the same time, cells are the smallest structures capable of performing the three vital functions of nutrition, interaction and reproduction. This is why cells are also known as functional units.
Diatom algae: an organism consisting of a single cell Elephant: a living thing made of many cells
As you can see, diatom algae and elephants are very different, so we divide living things into two categories:
- Unicellular: an organism consisting of a single cell, such as bacteria, fungi and algae.
- Multicellular: an organism consisting of more than one cell. All plants and animals are multicellular living things.
THE DISCOVERY OF CELLS: CELL THEORY
In 1665, the English scientist Robert Hooke first described a cell. He observed it using a rudimentary microscope which he had built himself. He observed a polygonal structure that was replicated multiple times inside thin sheets of cork. Hooke called this structure cella which means cell’ or gap’ in Latin.
In 1838, Matthias Schleiden and Theodor Schwann were the first scientists to formulate cell theory. This theory was later extended by other scientists, and nowadays it states that:
- All organisms are made of cells.
- Cells are the structural and functional units of living things.
- Every cell is the result of the division of a pre-existing cell.
- Cells are the simplest independent life units which we know of.
CELL STRUCTURE
Bacteria, skin cells and plant cells all have a basic structure, just like all cells found in nature. A cell consists of a plasma membrane, cytoplasm and at least one nucleic acid molecule.
- The plasma membrane is the envelope around the cell. It separates the inside of the cell from the exterior. It allows substances to enter and exit the cell. In addition to this membrane, some cells have another rigid outer casing which is called the cell wall.
- Cytoplasm is a watery substance found in the interiors of cells. In some cells, we can find organelles, which are specialised structures that perform specific functions. For example, a mitochondrion performs cellular respiration.
- The nucleic acid contains genetic information which is essential for the functioning of the cell. It can be dispersed within the cytoplasm, or surrounded by a membrane, which is called the nuclear membrane.
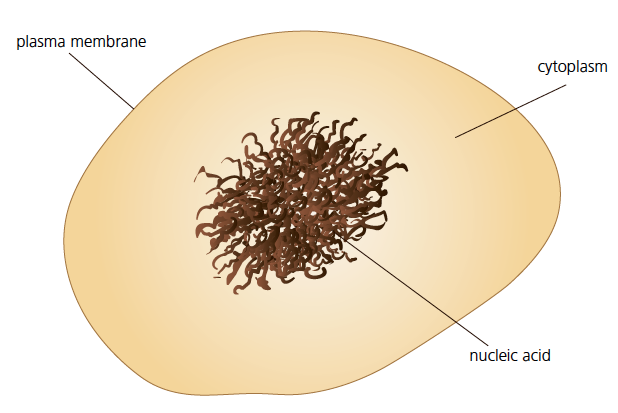
Cell size
Cells exist in different sizes, although generally they are microscopic. To measure cells we use micrometres (µm):
1 µm = 0.001 mm = 0.000001 m
1m = 1000 mm = 1000000 µm
CELL TYPES
Different species of living things have different amounts of cells as well as different cell structures. We can classify cells into two types according to their structures: prokaryotes and eukaryotes. Eukaryote cells differ according to whether they are found in animals or plants.
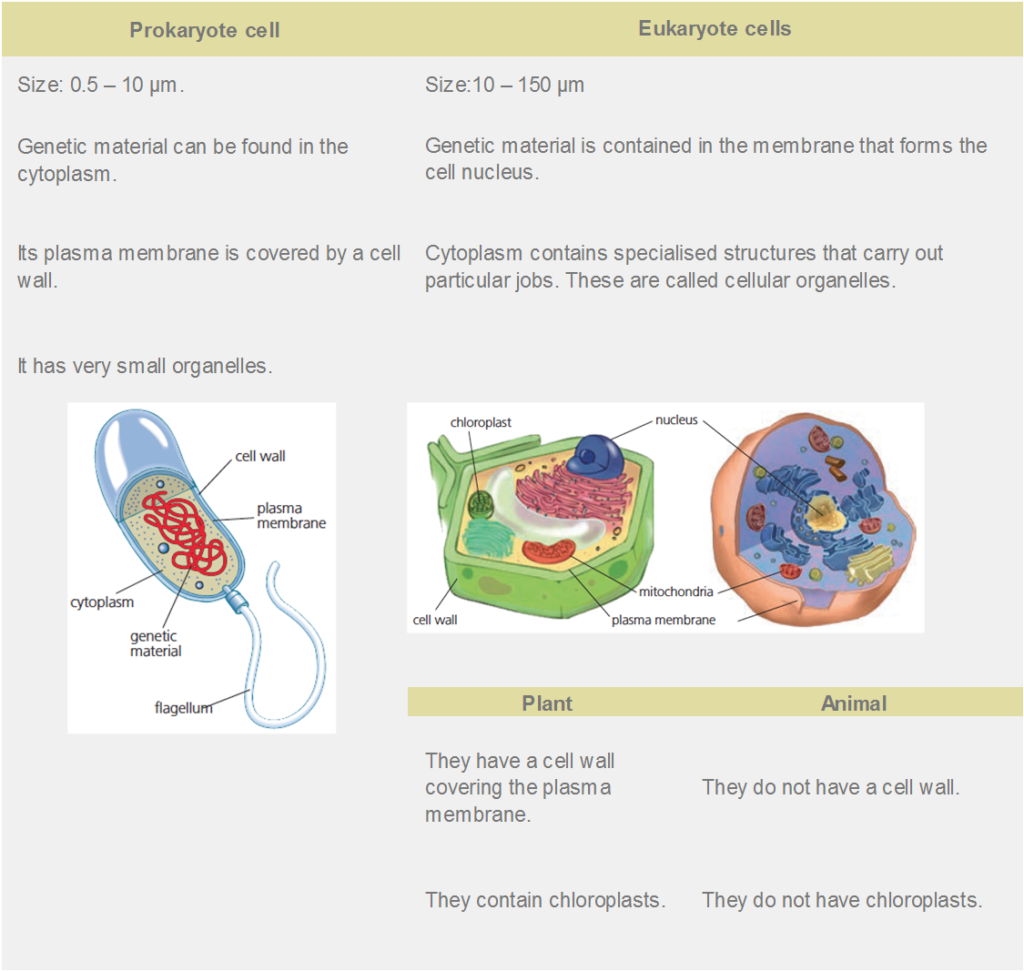
THE ENDOSYMBIOTIC THEORY
This theory was formulated by Lynn Margulis in 1967. It states that eukaryote cells originated from the interaction of several primitive prokaryote cells 500 million years ago.
Margulis proved that mitochondria and chloroplasts originated from primitive bacteria. Her theory is widely accepted by the scientific community.
CELL SPECIALISATION
Multicellular living things are made up of many cells. All cells in living things have a common characteristic: they have the same genetic material (DNA).
However, within the same organism, we can find many different types of cell. Each of them is specialised to perform a particular job.
Every type of cell uses one part of the information found in the DNA, as if the cell was reading a chapter of an instruction manual. This is known as cell specialisation.
Cell specialisation gives multicellular living things many advantages:
- Division of labour: Each type of cell performs a different job so living things can multitask.
- Greater efficiency: Because they do specific jobs, they do them very efficiently.
- Longevity: Damaged cells can be replaced by other cells. This means that multicellular organisms are more likely to survive.
However, multicellular living things need more energy to allow the different structures and functions to work at the same time.
Actividades
2. BIOELEMENTS AND BIOMOLECULES
Living things, like all matter in the universe, are made up of very small particles called atoms. These particles are so small you would not be able to see them, even if you used a microscope.
Atoms are the first structural level of matter and they organise themselves into molecules.
We know 117 different types of atom. Each of them constitutes a chemical element. A chemical element is a form of matter composed of atoms of the same type.
BIOELEMENTS
Bioelements are the units which living things are composed of.
The atoms that make up all living things mostly consist of six different chemical elements: carbon (C), oxygen (O) hydrogen (H), nitrogen (N), phosphorus (P) and sulphur (S). These elements constitute more than 99% of the matter found in living things. They are called primary bioelements.
Carbon atoms have the ability to link to other atoms. This allows life to take place and is why carbon is the most important bioelement.
Other chemical elements are found in living things; however, they appear in smaller quantities. We refer to them as secondary bioelements. They are: calcium (Ca), sodium (Na), magnesium (Mg) and potassium (K).
Lastly, trace elements are elements which are found in living things, but only in small amounts (0.1%). Although trace elements are not common, they are also important for certain functions. Examples of these are: iron (Fe) and iodine (I).
BIOMOLECULES
Bioelements join together to form different biomolecules. Biomolecules are what living things are made of.
Some biomolecules are exclusive to living things. They are known as organic biomolecules. These are: carbohydrates, lipids, proteins and nucleic acids.
Inorganic biomolecules (water or mineral salts) are also found in both living things and non-living things.
Water is essential for the existence of life and is the most common biomolecule. Between 50% and 95% of the weight of any living thing is water.
Percentage of water content of some living things
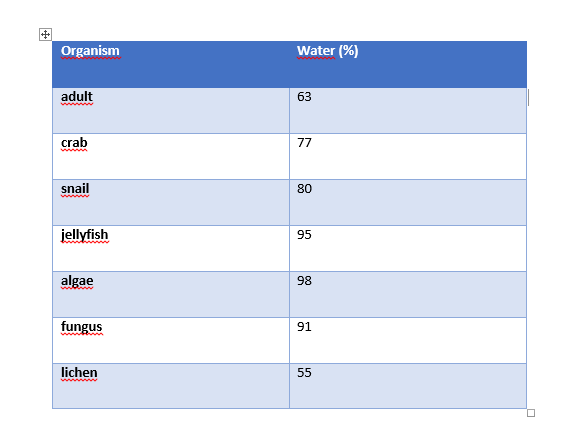
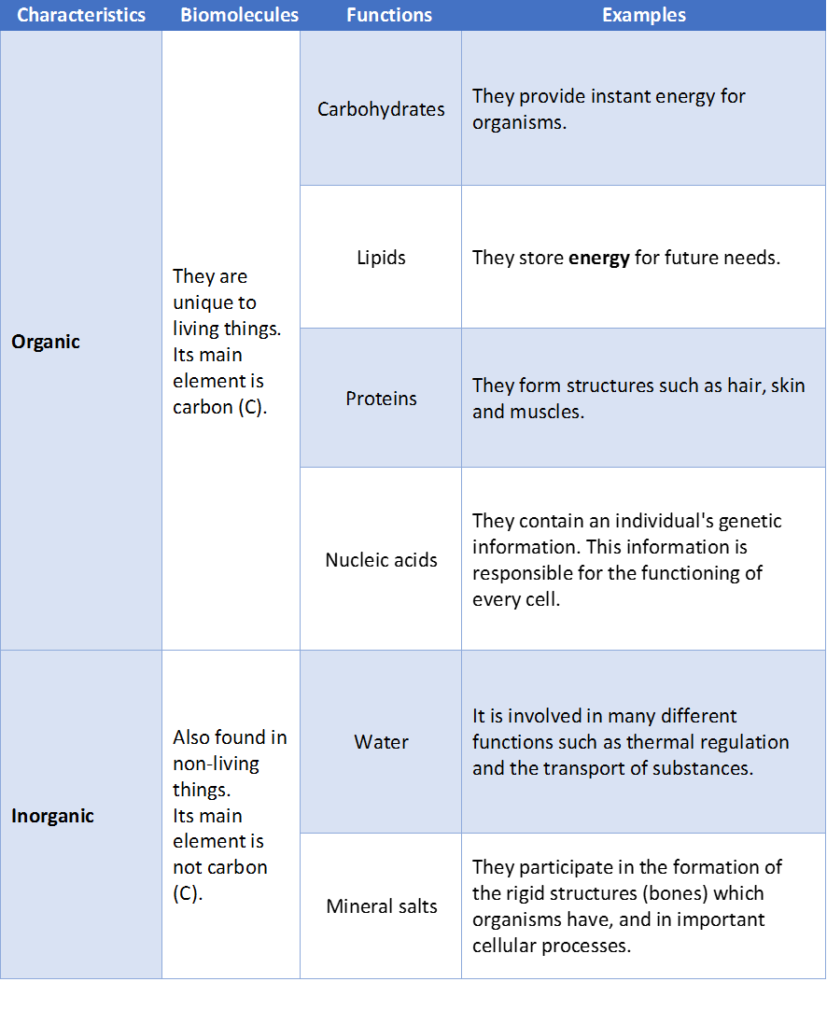
Actividades
Comentarios recientes